WELCOME TO THE GLASS LAB!
In the Glass Lab, we are interested in understanding how epigenetic signaling regulates gene expression, and how alterations in these pathways are involved in disease development, particularly cancer, heart, and infectious disease. We are investigating the molecular mechanisms driving the recognition of histone post-translational modifications in order to identify new therapeutic strategies.
The combinations of marks that make up the histone code have been difficult to decipher, and how multiple modifications modulate protein recognition is not well understood. We aim to determine how physiologically abundant combinations of histone modifications regulate chromatin reader activity to influence disease progression. To address our research questions, we use a diversity of approaches in molecular biology, genomics, biochemistry, biophysics, and proteomics.
The Glass lab is located in the Firestone Medical Research Building in the Larner College of Medicine at the University of Vermont. Dr. Glass is a faculty member in the Departments of Biochemistry and Pharmacology. Dr. Glass is also a member of the UVM Cancer Center, the Cardiovascular Research Institute, and the Vermont Center for Immunobiology and Infectious Disease. Follow the Glass lab on Twitter and Facebook!
For those interested in joining our lab, please note that scholarships are available for Ph.D. students.
Glass Lab current members:
Karen C. Glass, Associate Professor
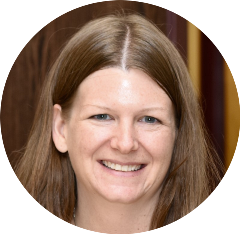
James Lignos, Ph.D. Student
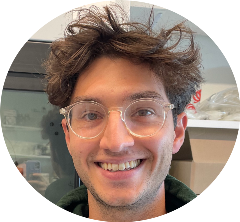
Bohan Liang, M.S. in Pharmacology student
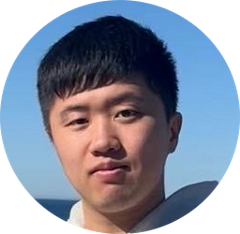
Annika Lothrop, Biochemistry student
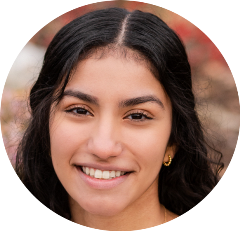
Ajit Singh, Postdoctoral Fellow
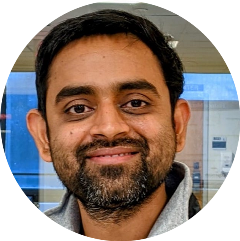
Kiera Malone, Ph.D. Student
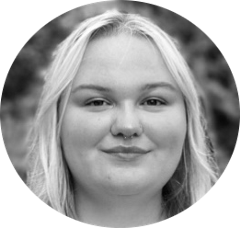
Elizabeth Cook, M.S. in Biochemistry student
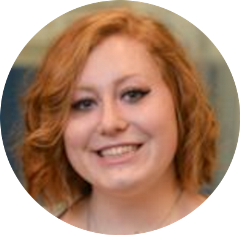
Glass Lab Research
Overview
Even though the DNA in all of our cells is
nearly the same, it is epigenetic factors, including modifications to DNA, RNA,
and chromatin, that control the gene expression patterns in different cells,
under various environmental conditions, and at specific times1. Post-translational modifications on histone tails function as a very
complex code for controlling gene expression. Deciphering this code is at least
as important as the genetic code for understanding its role in stem cell
programming, maternal effects during fetal development, and potential
reversibility in cancer therapies. However, it is also orders of magnitude more
complex2. We
study the molecular mechanisms driving the recognition of histone modifications,
and how combinations of these chemical signals regulate protein interactions on
the nucleus in both normal and disease states.
Major research efforts in the lab include:
·
To uncover how multiple chromatin reader
domains cooperatively regulate the activity of epigenetic regulatory complexes.
·
To reveal how cross-talk between epigenetic
marks modulates the function of histone binding proteins and chromatin
remodeling enzymes.
·
To identify and functionally
characterize how acetyllysine signaling and recognition contributes to the
pathogenesis of parasitic infections.
·
To correlate how altered epigenetic
modifications influence the progression of cancer, heart, and infectious
disease.
Background
The human genome is compacted into chromatin, allowing
nearly three meters of DNA to fit into the small volume of the nucleus.
Chromatin is composed of DNA and proteins, and this DNA-protein complex is the
template for a number of essential cell processes including transcription and
replication. Understanding the role of chromatin’s higher order structure in
transcriptional control is important as loss of this regulation underlies many
disease processes.
The basic structural unit of chromatin is the nucleosome.
Nucleosomes are comprised of 147 base pairs of DNA wrapped around a core histone
octamer. The histone octamer contains two molecules of each histone H2A, H2B,
H3 and H4. Each of these core histones contains two separate functional
domains; a globular domain, which interacts with the DNA and other histones,
and a flexible tail domain that protrudes from the nucleosome. The tail domains
can be modified by the reversible addition of chemical groups such as acetyl-,
methyl- and phospho- groups.
Modifications on the histone tail have been shown to be
important in altering chromatin structure, facilitating access for DNA-binding
transcription factors, but they also act as markers, allowing non-histone
proteins to interact with the chromatin. The “Histone Code Hypothesis” suggests
that histone tail modifications constitute an epigenetic (beyond genes) code,
which is read by other proteins. It postulates that these proteins, and protein
complexes are able to recognize/read distinct tail modifications, just like a
language or code. This consequently triggers downstream events resulting in
unique and specific biological outcomes such as: cell death, cell cycle
regulation, and the transcription, repair, or replication of DNA.
Recent Work
Epigenetic signaling by histone
post-translational modifications
We are investigating the structure and
function of chromatin binding domains, including the bromodomain, which
interact specifically with acetylated histones. There are about 60 human
bromodomain-containing proteins, and these nuclear proteins have a wide variety
of biological activities3.
Bromodomains bind to specific acetylation marks on the histone tail, and tether
associated proteins and enzymatic complexes to histones to regulate chromatin
structure and gene expression4. However, how
these protein modules differentiate between multiple acetylated lysine
residues, that are often found alongside other post-translational modifications,
to read the histone code is unknown. We recently established the molecular basis of histone
acetyllysine recognition by the ATAD2 and ATAD2B bromodomains and discovered
that theses bromodomains prefer to interact with histones
H4, H2A, and the H2A.X histone variant when they contain multiple acetylated
lysine residues. The structural and mechanistic details of histone recognition
by bromodomains is crucial for the development of new therapeutic
interventions and molecular tools to study a variety of diseases. Our research has fundamentally
advanced our understanding of how bromodomains recognize and select for
acetyllysine marks.
Insert: Figure 1
Figure 1: Multiple modifications on individual histone tails of the nucleosome
modulate the ATAD2/B bromodomain activity. In the
cell, ATAD2/B is localized at the chromatin, which is enriched with histone
PTMs. These modifications influence the ATAD2/B bromodomain activity. Adjacent
methyl groups negatively regulate the ATAD2 bromodomain's ability to recognize
the ‘Kac’ mark but do not significantly affect recognition of acetylated
histones by the ATAD2B bromodomain.
Bromodomain-containing proteins in cardiovascular and infectious diseases
Plasmodium
falciparum is a unicellular protozoan parasite that causes malaria
infections in humans. Malaria is a significant global health problem that
affected 247 million people in 2021, resulting in approximately 619,000 deaths5. Unfortunately, this
disease disproportionally affects infants and children, and the cases have been
expanding since 2016 due to climate changes and drug resistance5. In humans, P.
falciparum first replicates in the liver cells, and as the disease
progresses, it moves into the red blood cells (RBCs)6. The symptoms of
malaria are associated with repeated rounds of parasite replication, egress,
and invasion into the red blood cells7. At the red blood cell stage of infection, P. falciparum consumes
the RBCs hemoglobin, preventing it from carrying oxygen to the heart, which
results in anemic heart failure8. In addition, parasitized
RBCs stick to the wall of blood vessels in the heart and brain to evade the
immune system, which often leads to inflammation and causes blood vessel
blockage in these vital organs. These infection-related complications are directly
associated with the invasion of RBCs by the parasite. The P. falciparum
Bromodomain Protein 1 (PfBDP1) is a multi-domain nuclear protein that contains
a unique combination of ankyrin repeats (ANK) followed by a bromodomain (BRD) (Fig.
2A). In-vivo studies have demonstrated that association of PfBDP1
with acetylated chromatin at the promoters of invasion genes promotes their
expression, and knockdown of PfBPD1 strongly reduces expression, and blocks the
invasion of RBCs (Fig. 2B)9,10. The chromatin binding
activity of PfBDP1 is thought to play an essential role in the red blood cell
invasion process by controlling the expression of
genes that are required for cell entry. Thus, it is imperative to understand
the essential factors involved in the P. falciparum RBC invasion process
to develop therapeutic interventions.
Insert: Figure 2
Figure 2. Role of PfBDP1 in regulating the
expression of invasion related genes. (A) Domain organization of PfBDP1.
(B) (top) Recognition of acetylated lysine by PfBDP1 bridges it to chromatin
where it activates the transcription of
invasion related genes. (bottom) Knock-down of PfBDP1 prohibits this
interaction, preventing parasite invasion.
The focus of my research is aimed at determining the structures of chromatin binding domains, including the bromodomain and PHD finger, in complex with the histone tail to elucidate how histone tail modifications are recognized. This research will aid in a deeper understanding of how chromatin remodeling complexes are targeted to the chromatin and regulate gene expression. A greater understanding of how these molecular signaling pathways function and are regulated will provide insights into how they can be therapeutically manipulated, and may help to identify new diagnostic markers and targets to prevent and treat disease.
References:
- Time Magazine 172(2010).
- Nat Chem Biol 14, 206-214 (2018).
- Cell 149, 214-31 (2012).
- FEBS Lett (2014).
- https://www.who.int/publications/i/item/9789240064898, 2022).
- Cold Spring Harb Perspect Med 7(2017).
- Med Microbiol Immunol 201, 593-8 (2012).
- Hematology Am Soc Hematol Educ Program, 35-57 (2002).
- Cell Host Microbe 17, 741-51 (2015).
- Front Cell Dev Biol 10, 816558 (2022).